Food
Research
Green light is not fully absorbed by plant leaves and it is less efficient in driving photosynthesis when compared with red light. Therefore, some growers still believe that the green part of the spectrum in LED lights is not needed or is needed only for convenience of providing good colour balance of light and working conditions for visual inspection of plant health. However, the presence of the green light in a growth spectrum is shown to have a positive effect on yield, particularly when growing fruiting crops like tomatoes with multilayer canopy structure and long lifecycle.
Researchers at Wageningen University and Research (WUR) have published results from an experiment in which tomatoes were grown in greenhouse conditions under LED lights with only blue and red light in the spectrum or different percentages of green light mixed with blue and red (Weerheim et all 2019). The research demonstrated that grow lights containing 40% of green in the output spectrum increased yield of greenhouse tomato by 6%, when compared to tomatoes grown under supplemental LED light containing only blue and red light with the same total light intensity. WUR researchers also noticed that leaves in the middle layer of the canopy showed significant increases in the chlorophyll a:b ratio and carotenoid concentrations as the green light intensity increased. Those changes were not seen in top leaves and may indicate that a higher amount of light was available inside the canopy when green light was present in the supplemental grow light and spectrum.
To better understand how green light affects growth and light availability inside a dense tomato canopy, Heliospectra Plant Lab has grown tomatoes under four light treatments with similar light intensity and red to blue light ratios but different percentages of green light. Additionally, all light spectra contained far red (FR).
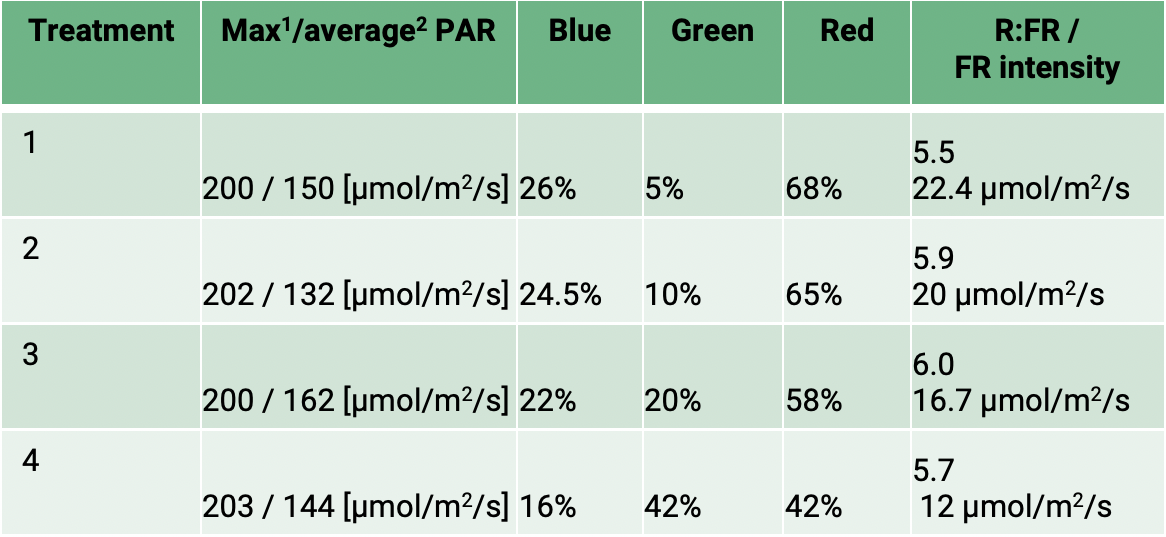
Table 1. Characteristic of light conditions in experimental units.
1Light intensity in the middle of the growth compartments at the pot height. The light intensity to which top leaves are exposed increases when plants grow taller. 2Average light intensity is equal to total light available for plants growing in the compartment.
Results and discussion:
Size of ripe fruits increased with the percentage of green light:
Total plant biomass and fruit biomass at the end of the experiment were somewhat affected by differences in pruning between treatments but not by composition of grow light spectrum. Plants in the treatment with the lowest green light percentage (5%) and the highest green light percentage (40%) had the same number of fully developed leaves (i.e. they were pruned in the same way), and they had almost identical biomass of stems, leaves and total fruit. However, plants grown under 40% green light tended to have higher percentage of large, ripe fruits at the end of the experiment. On average 90% of ripened fruits from plants grown under light treatment with high green percentage were of A class or categorized as large size and only. 70% from plants grown under 5% green treatment reached full size and were categorized a large. Fruits of plants grown under 40% green light were also 18% heavier on average than fruits of plants grown under the 5% green light treatment (fig. 2). The average number of tomatoes per truss did differ significantly (7.6 vs. 7.4 tomatoes per truss, respectively). However, the tomatoes grown under 40% green light had fewer trusses with developing fruits and more with flowers.
The results of this experiment indicate that the total biomass of the plant (I.e.stem leaves and fruits) depends on the total amount of light available to the plant, regardless of the light spectrum. And, the increasing proportion of green light in the grow light spectrum may encourage better fruit development.
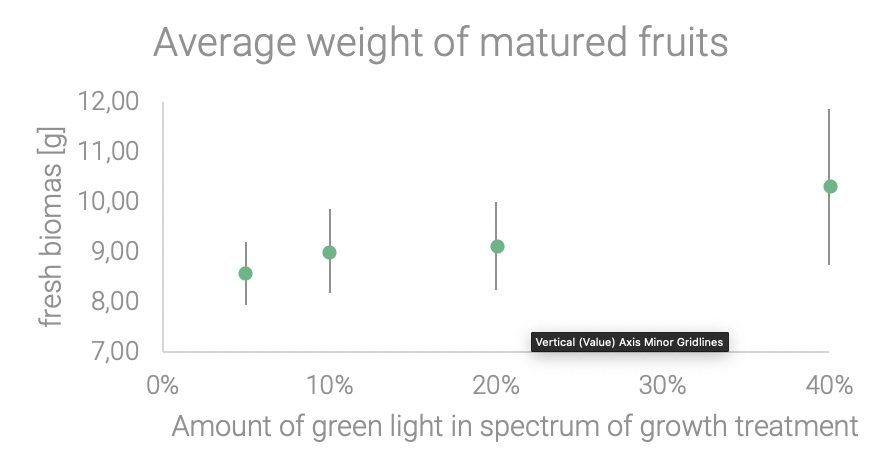
Figure 1. Average weight of mature, ripe and ripening tomatoes at the end of experiment was measured as a total weight of mature tomatoes per plant divided by their number. Error bars represent standard deviation of average weight between plants. N=3 plants per treatment.
Green light in grow light spectrum increases availability of PAR light inside the plant canopy:
Light availability inside the dense tomato leaf canopy decreased exponentially as the distance from the top of the canopy increased. Measurements inside the canopy were compared with the light intensity inside the growth compartments without plants. Therefore, the results were affected by leaf shading and did not depend on the distance from the LED grow lights. Light intensity measurements varied substantially at different points within the canopy, yet, on average more light was available at the deeper leaf layers of plants grown under 40% green light treatment.The differences between the 40% and 5% green light treatments were significant at most of the measurement points (fig 2). It appears that most of the light available at deeper layers of the canopy comes through the openings between the leaves and not from light transmission through the leaves as the proportion of green, blue and red light did not differ substantially with increases of FR from the growth spectrum. The green light ratio was increased at very low light levels (data not shown).
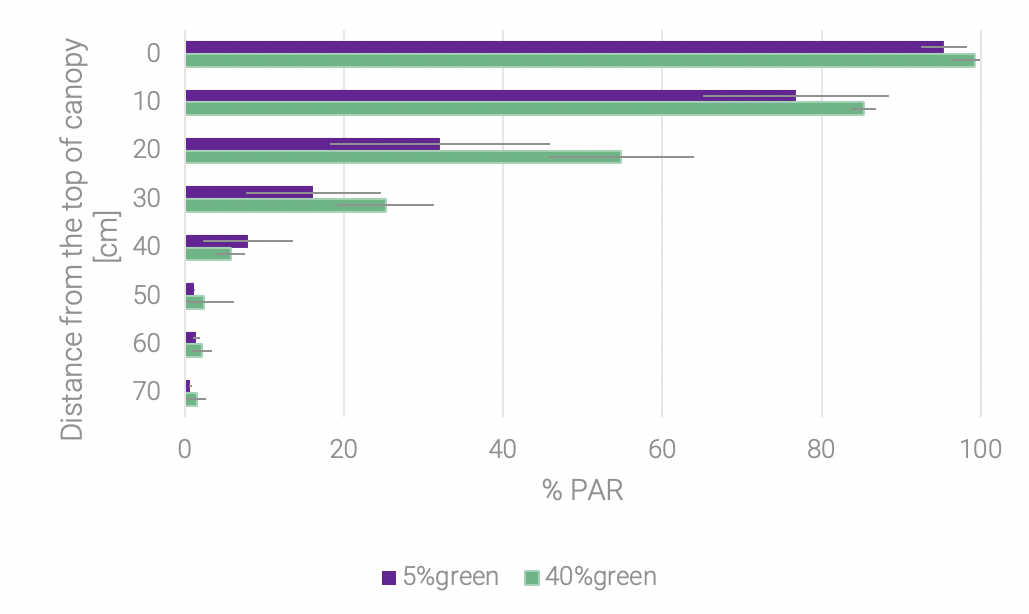
Figure 2. Comparison of PAR light intensity available inside a dense tomato canopy grown under spectra with5% and 40 % green light treatments, shown as percentage of light measured at the same height in a growth compartments without plants. Bars represent standard deviation N=3 measurement series per treatment.
The amount of the light passing through the leaves (transmittance) depended on the amount of light the leaf was exposed as well as the grow light spectrum. Leaves of tomato plants grown under 40% green light had up to 5 times higher transmittance as those grown plants grown under 5% green light. The photons, mostly green and far red, filtered by the top leaves can be still used to provide energy for lower leaves situated inside the canopy even if they are not fully absorbed. However, in the case of tomatoes, the transmittance of light through the leaves is low and values obtained in this experiment were under 3% of incoming light (data not shown).
The presence of green light in grow light spectrum affect plants architecture:
Plants grown under the higher percentage of green light had tendency to display wider and longer leaves (fig 3), with larger spaces between leaflets. Such architecture allows more light to reach to the deeper layer of canopy.
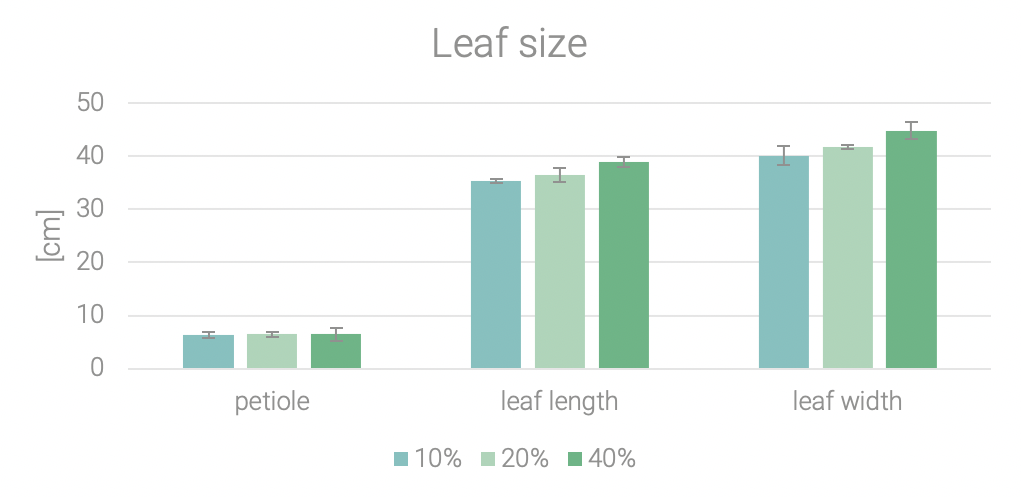
Figure 3. Average size of leaves #3 to #8 under light treatments with different percentages of green light. Leaves under 5% green light were not measured. Error bars represent average standard deviation from 3 plants per treatment.
The effect of far-red (FR) light:
Although all light treatments were set to have a similar R:FR ratio, the percentage of FR light in the spectrum decreased as the amount of green light increased. A larger portion of red light was substituted by green due to the increasing mixture of green light. Observed changes in fruit size (fig. 1) and leaf morphology (fig. 3) are opposite to the known effects of FR. FR is responsible for elongation of stems, petioles and other leaf parts (reviewed by Demotes-Mainard et al 2016). Additional FR was also reported to increase the biomass allocation to tomato fruits (Ji et al 2019). Therefore, differences observed by Heliospectra between light treatments are more likely to be caused by green light than additional FR light.
Optimal solar light spectrum:
Green light constitutes about 35% of the photosynthetically active radiation of sunlight (Chiang et al 2019). There is growing evidence showing that green light percentages similar to this level might be optimal for radiation capture by the whole plant, which consequently leads to high productivity and yield (Kaiser 2019). In a situation where the light is very strong, green photons have a greater potential to drive photosynthesis because they can penetrate further into the leaf tissue than red or blue photons which are absorbed mostly at the surface of a leaf (Nishio 2000, Terashima et al2009).
A similar principle can be applied to the whole plant canopy profile (Smith et al 2017).In strong light with a low proportion of green light or when only blue and red light is present, the photosynthetic apparatus of leaves at the top of the canopy will be saturated while leaves inside the canopy may not receive enough light to sustain effective photosynthesis. When the amount of blue light exceeds the amount of green light (a high B:G ratio), many plant species increase the production of anthocyanins which are pigments working as a sunscreen for the leaves. Anthocyanins absorb a portion of PAR light energy, which then does not contribute to photosynthesis. This mechanism has a very important function of protecting the photosynthetic apparatus from damage by strong light (Gould et al 2008), but when it is triggered by non-saturating light spectrum composition, the production of anthocyanins may also limit the efficiency of light harvesting. An excess amount of green in a spectrum (or low B:G ratio) triggers shade response and may negatively affect plants by promoting excess elongation and reducing biomass accumulation (Zhang et al 2011).
Differences between laboratory conditions and a greenhouse:
In the laboratory condition the total amount of light potentially available to the plants depends only on the amount of light produced by the grow lights. As non-saturating light intensity and plant canopies fill all available space, it can be expected that plants are harvesting virtually all available photons. The quality of the grow light spectrum in such conditions can affect the proportion of light harvested at different layers of leaves(i.e. less photons are harvested on the top and inside of canopy), but not the total amount of the light available to a plant.
Ina greenhouse, plant structure is mostly affected by solar light. Effects of artificial lighting in a greenhouse is visible during the seasonal part of the year with low availability of natural light. Even small differences in plant structure can affect light levels inside the plant canopy and may influence the total leaf area that is active in light harvesting, thereby affecting plant biomass and yield. Also, it can be expected that changes in light penetration may directly affect fruit growth and maturation directly, since small green fruits can photo synthesize and sustain a part of their own energetic needs when sufficient light is available.
Do I need green diodes in my LED lamp?
Performance of green LED diodes are still unsatisfactory when compared with LEDs of other colours However, the green part of the spectrum can be provided by using white LEDs which are more efficient.White LEDs are blue diodes, with peak emission in the blue region, coated with a layer of phosphor. The phosphor coating converts blue light to a broad light band covering green and red wavebands of the spectrum. A part of the blue light transmits through the coating, so such diodes produce a mixture of blue, red, and green photons, and their light output is perceived by humans as white light. To increase fixture efficacy and create optimum light spectrum for plants, the white diodes must be accompanied by red, and depending on fixture application, also by blue and far red diodes.
Heliospectra has designed its new MITRAlight to combine properties of natural solar light with the high efficacy ofLEDs. MITRA lights have a spectrum with 40% green light to promote efficient light interception by plants and deep penetration of light inside the plant canopy which can have a positive effect on tomato yield and fruit quality. At the same time, the MITRA spectrum contains enough blue light to prevent excess elongation, assure balanced photosynthesis in the top leaves, and stimulate production of secondary metabolites. In comparison to traditional lighting such as horticultural HPS, metal halide, or fluorescent tubes which also provide broad spectrum white light, MITRA has at least 35% higher efficacy1. (1When compared to new, 1000W HPS bulb.)
Methods:
Dwarf, bushy variety of cherry tomatoes Solanum lycopersicum ‘Romello‘,were grown under four light treatments with similar PAR light intensity and red to blue light ratios but different percentages of green light. All light spectra treatments contained far-red (FR) adjusted to provide similar R to FR ratio in all light treatments. Treatments with 5% 10% and 20% of green were set using ELIXIA adjustable spectrum fixtures while the 40% green treatment was provided with the MITRA fixed spectrum light. Growth compartments were lined with a black fabric to reduce light reflection from walls and block additional light to lower parts of plant canopy. Temperature, humidity, and air flow were monitored throughout the experiment and were similar in all growth compartments.
Tomatoes were moved to growth compartments situated in a climate-controlled room as seedlings with first two leaves developed. In the beginning of the experiment nine tomato seedlings were grown in each 1.2 m x1.2 m unit. As the canopy was closing, the plant density was reduced to six and then three plants.After five and seven weeks in the experiment, three plants were removed. The remaining three plants were tied and allowed to grow for another 4.5 weeks. At the beginning of treatments, the tomatoes were pruned regularly. During the last month of the experiment, branches were left unpruned to create a dense canopy and let the plants fill the whole available space in the growth compartments.During the pruning procedure, lower leaves and excess branches were sequentially removed to leave two main stems per plant. In the process, one of the main branches of a tomato plant under10% green light treatment was accidentally broken resulting in lower average biomass and fruit set. No flowers or fruits were removed from growing trusses. The experiment was concluded after 80 days. Stems, leaves and fruits of tomato plants were weighed and leaves from the third to eighth leaf were measured.
Light intensity inside the canopy and light transmittance through the leaves were measured before harvest with an Ocean Optic JAZ spectrometer. Light intensity was measured with an optical fibre at straight vertical line at 7 points inside of canopy and compared with a similar measurement done in each chamber after removing plants. Transmittance was measured in situ as a proportion of intensity of incoming light from the industrial LED grow light and of the light under the leaf placed in the optic fibre adhered with a black metal block.
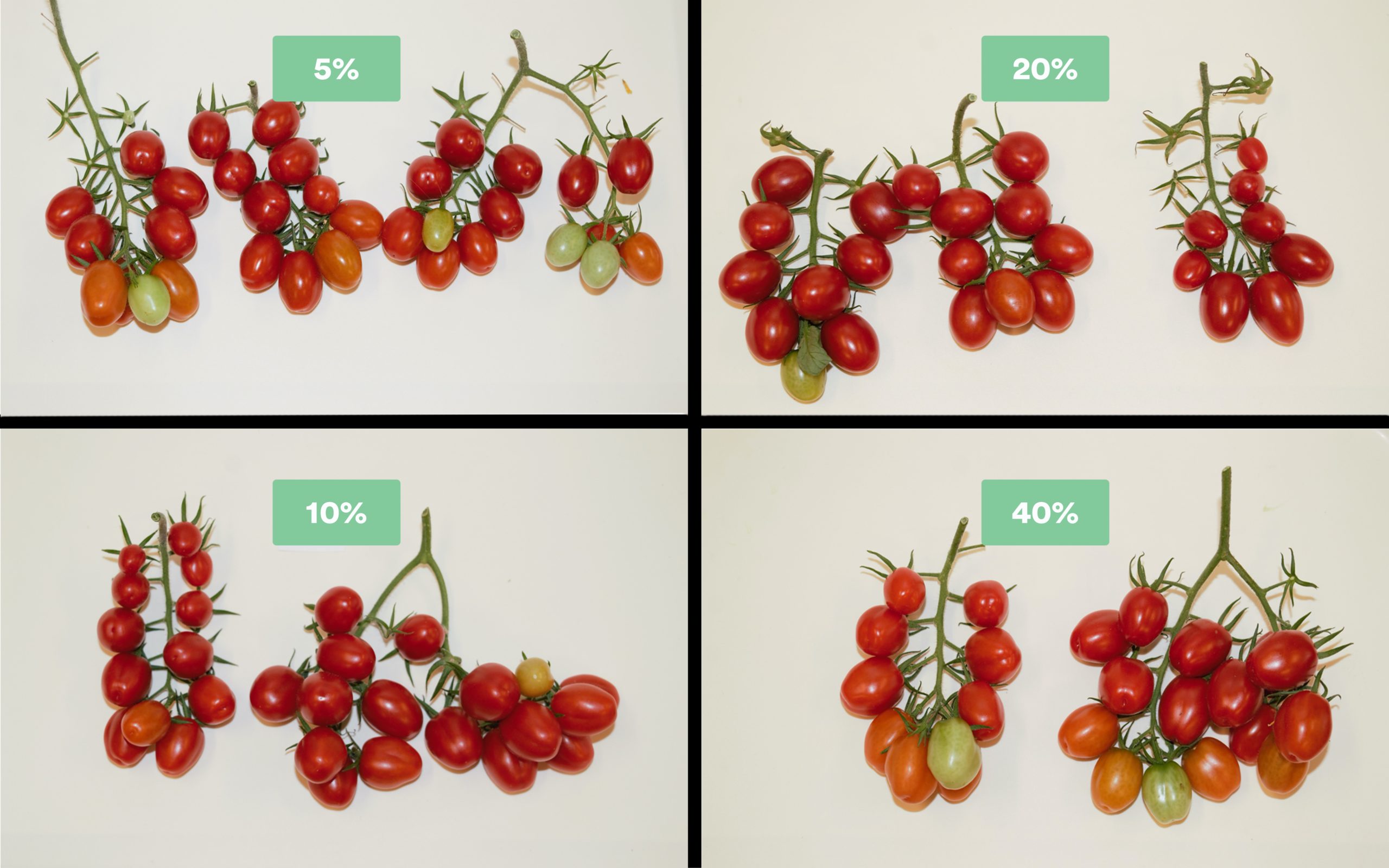
Figure 2. Trusses of tomatoes at the harvest. Plants under 40% of green produced larger and more uniform fruits.
Literature
Chiang et al, 2019 https://doi.org/10.3390/f10080610
Demotes-Mainard et al, 2016 https://doi.org/10.1016/j.envexpbot.2015.05.010
Gould et al, 2018 https://doi.org/10.1016/j.envexpbot.2018.02.006
Ji et al 2019 https://doi.org/10.1016/j.envexpbot.2019.103889
Nishio 2000, https://doi.org/10.1046/j.1365-3040.2000.00563.x
Terashima et al, 2009 https://doi.org/10.1093/pcp/pcp034
Smith et al, 2017 https://doi.org/10.1093/jxb/erx098
Weerheim et all, 2019 https://doi.org/10.1016/j.scienta.2019.02.005
Zhang et al, 2011 https://doi.org/10.1104/pp.111.180661
Related content
Get in touch with us!
From custom light planning, to tailored quotes, and everything in between,
our team of horticulture experts are always ready to assist.
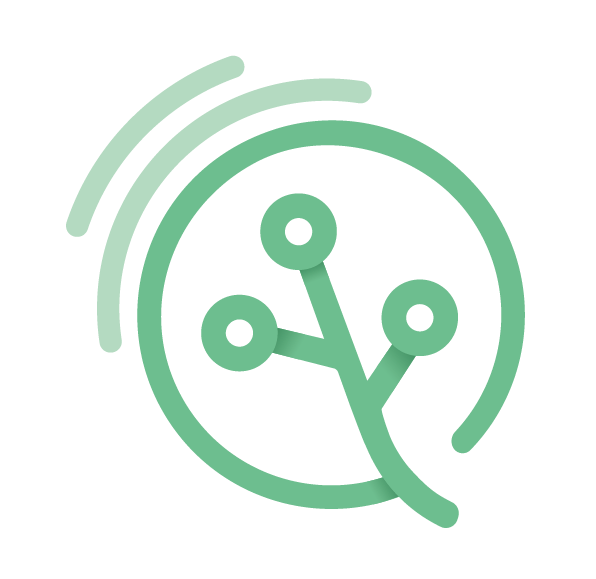